Particle Physics
Fermilab’s science strategy enables a diverse science program with major discovery potential, from the science of neutrinos and the Large Hadron Collider, to the search for dark matter and anomalies in precision science, to the study of dark energy. To realize this strategy, the laboratory is advancing major initiatives in neutrino science, LHC science, precision science, cosmic science, and quantum sensors. The overarching goal is to maintain and enhance a world-leading program that serves the needs and scientific goals of the U.S. and international particle physics community.
Neutrino Science
The revolutionary discovery that neutrinos can change from one type to another—and hence have mass—sparked a global initiative to investigate the phenomenon known as neutrino oscillations. The scientific community’s vision for the future of U.S. particle physics is laid out in the P5 report and envisions Fermilab as the most advanced and diverse accelerator-based neutrino facility in the world. The community will achieve this ambitious goal by completing major upgrades to Fermilab’s accelerator complex to deliver megawatt particle beams, building a new suite of three on-site neutrino experiments that will also serve as a test bed for advanced neutrino detectors, and leveraging international partnerships to construct the flagship international LBNF/DUNE facility. In 2015, Fermilab identified the need to establish a neutrino user community with more than 1,000 members as a 10-year goal. This goal will be achieved 7 years ahead of schedule, with the DUNE collaboration alone now numbering 950 scientists from 30 countries and expected to pass 1,000 members within the next year.
Fermilab is the one facility in the world that simultaneously operates two accelerator-based neutrino beams. These two intense beams drive an ensemble of experiments that are studying the detailed properties of neutrinos at both low and high energies and over both short and long distances. Updated results from the long-baseline NOvA experiment, based on 16% of its initial planned data set, disfavor at more than 2.5 sigma a muon-tau flavor symmetry in neutrino mixing. NOvA results also disfavor combinations of an inverted neutrino mass hierarchy, lower values of the so-called atmospheric mixing angle, and CP violation effects that would suppress the appearance probability for electron neutrinos. The success of current and future neutrino experiments depends critically on beam delivery. Accelerator improvements underway now, and even more ambitious upgrades planned for the next decade, will guarantee Fermilab’s pre-eminence as the world’s source of accelerator-driven neutrino beams for the next 20 years. The first of these upgrades, the Proton Improvement Plan (PIP), has doubled the number of protons used to make low-energy neutrino beams and increased proton beam power for high-energy neutrino experiments to 700 kW. The next accelerator upgrade (PIP-II) will replace the lab’s existing 40-year-old linear accelerator with a new one based on superconducting RF cavities. PIP-II will increase the proton beam intensity for high-energy neutrino experiments to 1.2 MW by 2025, and will provide a platform for a future increase of the proton beam intensity to 2 MW for experiments envisioned beyond 2025. Fermilab is also proposing a series of upgrades, PIP-I+, to achieve up to 1 MW by upgrading the existing accelerator complex prior to PIP-II.
For the short-baseline neutrino program, a three-detector suite of experiments being built on the Fermilab site has the potential to make a groundbreaking discovery: the discovery of a fourth type of neutrino, the so-called sterile neutrino. An international team is preparing three detectors based on liquid-argon time-projection-chamber (TPC) technology with the goal of bringing all three online by 2019. Besides definitively addressing time-critical physics, this program will also provide an important platform for detector development and international coordination towards LBNF/DUNE. The first in this succession is the MicroBooNE experiment, using a 170-ton detector that began taking data in 2015. While pushing towards significant advances in the liquid-argon TPC technology, MicroBooNE will also produce the first measurements of low-energy neutrino interactions in argon. By 2019, two additional detectors will have come online: the Short-Baseline Neutrino Detector (SBND) that will be closest to the neutrino source; and the ICARUS T600—the largest liquid-argon TPC in the world— that has been refurbished at CERN and will be transported to Fermilab in 2017.
Although the future short- and long-baseline neutrino programs involve multiple scientific collaborations that have distinct scientific goals, synergies in liquid-argon TPC technology and personnel provide coherence. The two programs together provide a coordinated approach to addressing some of the most fundamental questions about neutrinos, but also provide a means to grow the technical expertise necessary to achieve success. The integrated program managed and enabled by facilities and staff at Fermilab will provide groundbreaking opportunities in research while also developing the expertise, technology, and computing required for the long-term program.
The ultimate goal of Fermilab’s neutrino science strategy is the operation of the LBNF/DUNE program. This new large-scale user facility is a major initiative and is described in more detail in the LBNF/DUNE section of the strategic plan.
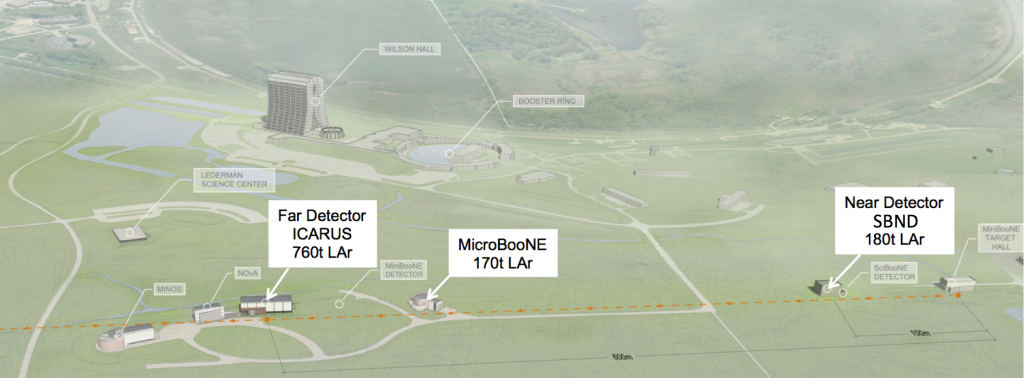
The new international short-baseline neutrino program at Fermilab that will include three liquid-argon (LAr) detectors on the Booster neutrino beam line: SBND at 110m, MicroBooNE at 470m, and the ICARUS T600 detector at 600m from the Booster neutrino source.
LHC Science
The Large Hadron Collider (LHC) at CERN is currently the only machine that produces the Higgs boson in sufficient quantities for study, and is a critical component of the multifaceted explorations of dark matter and the unknown—three of the key science drivers identified in the P5 report. Fermilab’s four core capabilities allow the laboratory to play a significant role in driving LHC science over the next ten years through leadership roles in the CMS collaboration and technological contributions to the LHC accelerator and CMS detector upgrades. Two phases of upgrades, including the ongoing LHC CMS detector upgrade and the upcoming detector and accelerator upgrades for High Luminosity LHC (HL-LHC) operation, were identified in the P5 report as the U.S. community’s highest-priority near-term large project. Looking farther ahead, Fermilab plays critical roles in carrying out long-term R&D for accelerator technology and the physics program of future colliders.
Fermilab’s continued participation in the international CMS experiment will ensure the collaboration’s scientific success throughout LHC Run 2 (2015-2018), Run 3 (2021-2023), and into the HL-LHC era (beginning in 2026). Scientific leadership for the CMS experiment is currently being provided by Joel Butler, a Fermilab scientist elected as spokesperson for the 3,000-member CMS collaboration in 2016. The laboratory also provides scientific leadership through contributions of its approximately 60 scientists to publication of innovative analyses of CMS data, technical and managerial contributions to detector upgrades and operations, and maintenance and continuing development of computing facilities and software infrastructure.
Fermilab has proposed making the CMS Center a DOE/SC national user facility that promotes U.S. scientific leadership at the LHC by supporting over 1,000 users through its LHC Physics Center (LPC), LHC Remote Operations Center, and dedicated computing facilities. In this context, the LPC already provides a vibrant on-shore academic environment focused around the LHC that includes tutorials, seminars, colloquia, visiting theorists, and organized and informal discussions. The annual LPC-hosted CMS Data Analysis School is crucial for training the newest members of the U.S. particle physics community, and the LPC Distinguished Researcher program is critical for maintaining a core group of physicists with diverse expertise at the LPC. The proposal to make the CMS Center a national user facility is currently under review by DOE/SC.
Precision Science
Experiments that study the properties of muons with high precision have the potential to elucidate the physics that leads to a low-mass Higgs boson, indicate the existence of new dark sectors responsible for dark matter, shed light on non-standard neutrino interactions, and indirectly probe energy scales as high as 104 TeV. Muon physics was identified by the P5 report as a critical component of the science driver to “explore the unknown” and the strategy correspondingly endorsed the construction of a Muon Campus at Fermilab that includes a new muon beam and two forefront experiments. This year the Muon g-2 experiment will begin using the new muon beam to carry out the highest-precision measurement of the anomalous magnetic moment of the muon and by 2021, the Mu2e experiment will be searching for charged-lepton-flavor violation using muons. The growing precision science program currently hosts 385 users from 57 U.S. and international institutions.
To realize the vision of a Muon Campus, Fermilab has constructed the world’s brightest muon source, has refurbished the Muon g-2 storage ring into a first-in-class technical instrument, and is building the Mu2e project. The new muon source, to be commissioned this year, will eventually deliver a muon flux to the Muon g-2 experiment that is 20 times greater than that achieved for the previous experiment at Brookhaven National Laboratory. The refurbished Muon g-2 storage ring will allow for a measurement of the muon’s magnetic moment that is a factor of four more precise than previously achieved. The Mu2e apparatus will allow for a factor of 10,000 improvement in the search for charged-lepton-flavor violation. Building and operating these facilities brings together expertise from particle, nuclear, and atomic physics. Fermilab staff designed most aspects of the technical instrumentation for Muon g-2 and Mu2e, and through their leading roles in the two experiments’ scientific collaborations defined the physics program of the Muon Campus. The laboratory’s strong lattice gauge theory group is deeply embedded in improving the Standard Model calculation of Muon g-2 so that the precision in the expected value will shrink in relation to the expected improved precision in the experimental value.
Fermilab’s accelerator science and technology core capability includes the ability to develop technologies to accelerate, characterize, and manipulate the particle beams that are required for precision science. For example, experimental, computational, and theoretical research on the physics of particle beams has been important in designing beam delivery systems for Muon g-2 and Mu2e. The configuration of the Fermilab Accelerator Complex is unique in its ability to simultaneously deliver beam to the muon and neutrino programs, and the PIP-II upgrade will support a factor of ten enhancement in beams delivered to a possible second generation Mu2e experiment. Fermilab’s core capability in advanced computer science, visualization, and data is critical to the success of this program since the multi-petabyte data sets are orders of magnitude larger than those generated by the previous iterations of these experiments.
The Muon Campus is a significant addition to Fermilab’s scientific user facility and is in close proximity to the Central Campus. The Muon Campus program advances the mission of the DOE Office of High Energy Physics with the ability to deliver significant and potentially revolutionary results. The program has helped foster integration by driving closer collaboration with Brookhaven, Argonne and Lawrence Berkeley national laboratories, with the NSF through a Major Research Instrumentation grant to provide the detector package for Muon g-2, and with seven other nations to optimize the use of the resources available in achieving our scientific goals.
Cosmic Science
The P5 report identified the new physics of dark matter, understanding cosmic acceleration, and pursuing the physics of neutrinos as three of the primary science drivers in particle physics, each of which is addressed by the study of cosmic science. With its strong particle astrophysics program and advanced infrastructure, Fermilab is a leader in all of these areas and an active participant in the DOE Cosmic Visions planning process. Over the next decade, the laboratory will continue to explore cosmic acceleration through large-scale surveys, build the next generation of detectors for the cosmic microwave background, and advance the search for dark matter using select technologies.
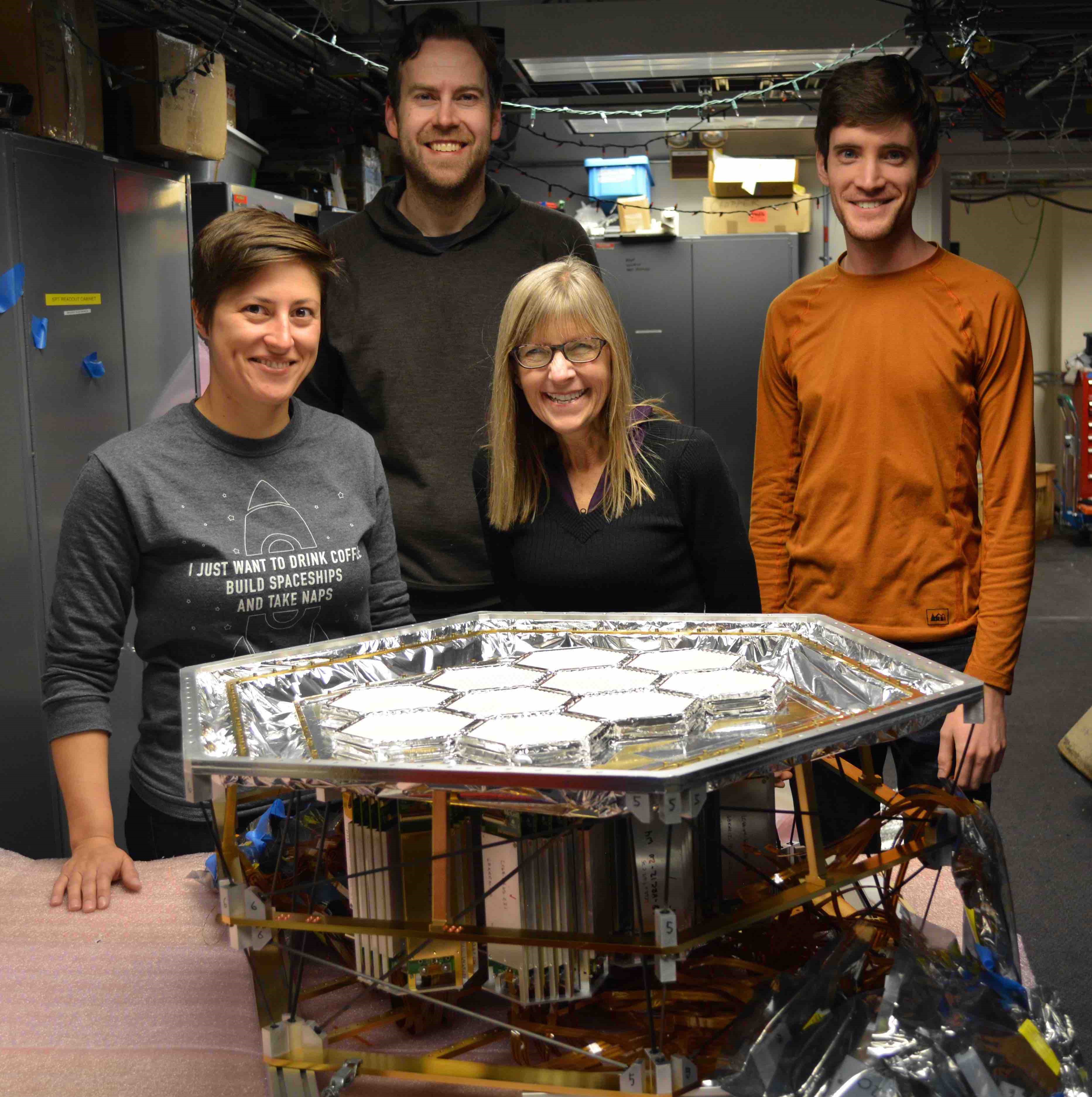
The Fermilab CMB team in front of the fully assembled SPT-3G focal plane at the South Pole, Antarctica. Pictured from left to right: Alexandra Rahlin (postdoc), Bradford Benson (scientist), Donna Kubik (physicist), and Adam Anderson (postdoc).
The Dark Energy Survey (DES), which recently finished its fourth year of data taking, is the world leader in exploring the mysterious phenomenon of dark energy. Fermilab both manages operations and leads analysis of data from this forefront survey. Over 100 scientific papers have been submitted and 83 published so far. Many of these papers covered the groundwork needed to extract the full potential of the DES data; new cosmology results are expected in 2017. Fermilab scientists led the analysis that placed tight constraints on intermediate mass primordial black holes as a dark matter candidate and have built frameworks that enable the collaboration to go from catalogs to cosmological constraints. These frameworks are being used in the analysis that will be released in Spring 2017. The first cosmological results from DES supernovae are also expected in 2017. These results will use several new techniques, including a deep learning technique invented at Fermilab.
Over the next ten years, Fermilab will play important roles in two cosmic surveys strongly endorsed by P5, the Dark Energy Spectroscopic Instrument (DESI) and the Large Synoptic Survey Telescope (LSST). For DESI, Fermilab will provide scientific management, a massive image corrector system, the online database, software to ensure that DESI observes the correct targets, and detector packaging and testing. Because of its experience leading the Sloan Digital Sky Survey and DES, Fermilab has a unique understanding of the challenges facing LSST and will be an active participant in the LSST Dark Energy Science Collaboration. The laboratory is currently developing a software framework for dark energy science at LSST based on the one used by DES, leveraging Fermilab’s core capability in advanced computer science.
Motivated in part by ideas generated by the Fermilab Astro Theory Group, the Cosmic Microwave Background (CMB) has become the most precise tool for understanding cosmology, with significant implications for particle physics. The P5 report endorsed DOE participation in CMB-S4, a U.S.-led program to build a next-generation CMB experiment and enable new constraints on the physics of inflation, neutrinos, and dark energy. Fermilab plans to become a leader in CMB studies over the next ten years.
Building off expertise developed for DES, Fermilab led the design, fabrication, and integration of the new SPT-3G camera for the South Pole Telescope (SPT). This fall, the SPT-3G camera was completed on schedule and shipped to the South Pole. The Fermilab team spent months at the South Pole installing and commissioning the SPT-3G camera, which will improve the mapping speed of the SPT by more than a factor of ten. A recent Laboratory Directed Research and Development (LDRD) award has allowed Fermilab scientists to begin developing next generation CMB detectors and instrumentation, positioning the laboratory for a leading role on CMB-S4.
Fermilab is committed to understanding the nature of dark matter and plays a key role in the second-generation (G2) dark matter projects now underway. Over the next several years, Fermilab will co-lead the SuperCDMS-SNOLAB project with SLAC National Accelerator Laboratory, building on world-leading limits on low-mass dark matter released in the past year. Fermilab is also a strong contributor to the LUX-Zeplin (LZ) experiment at the Sanford Underground Research Facility, providing cryogenic and process control engineering to safely manage the 10 tons of liquid xenon required by the detector. The LZ experiment will improve on the current best sensitivity for high-mass dark matter by a factor of a hundred. Rounding out the G2 program, Fermilab is the DOE lead lab for the operations phase of the Axion Dark Matter Experiment (ADMX) that searches for axions that scatter in a strong magnetic field and convert into microwave photons. Also, supported in part by LDRD funding, Fermilab’s core capability in accelerator science and technology will be used to study high-quality-factor resonant cavities and single microwave photon detectors, enabling future high-mass axion searches.
Fermilab’s detector R&D program has supported many efforts in cosmic science. Fermilab led the development of the COUPP/PICO bubble-chamber dark-matter detectors and leveraged its DES experience to pioneer the use of Charge Coupled Devices (CCDs) as low-threshold detectors in the Dark Matter in CCDs (DAMIC) experiment, with both efforts leading to recent world-leading results. The DAMIC effort, which was supported by a Presidential Early Career Award for Scientists and Engineers, and the PICO effort grew into international collaborations. Leadership of the DAMIC collaboration was recently transferred from Fermilab to the University of Chicago to focus Fermilab’s efforts on the G2 dark matter program. Similarly, leadership of the PICO collaboration was recently moved from Fermilab to Canada and Pacific Northwest National Laboratory. Fermilab researchers supported by a 2016 LDRD award developed even lower-noise CCD readout, enabling the detection of single electrons and opening a potential new window for testing dark photon models of dark matter. Fermilab will continue R&D towards next-generation detectors for cosmic science, for example developing cryogenic systems for superconducting detectors with sub-Kelvin capabilities.
Cosmic science provides a showcase for the benefits of broad collaboration among DOE laboratories and universities. As noted in the P5 report, particle physics drivers are intertwined, and cross-project expertise is required to extract the most science from the data. Fermilab scientists are leading these efforts. For example, the dwarf galaxies discovered by DES have led to dark matter constraints, and joint analyses of the DES and SPT data sets have placed new constraints on dark energy and gravity at large scales. Over the next decades, Fermilab will act as a central platform and host for understanding cosmic science data, maximizing the scientific output of experiments across the field.
Quantum Sensors
Research into quantum sensors provides an opportunity for cross-fertilization and technology transfer between the field of particle physics and the field of quantum computing. Quantum computing relies on the accurate manipulation of qubits of information encoded using single microwave photons. The high fidelity required for quantum computing translates into high quantum efficiency and low dark rates when these qubit devices are repurposed as single-photon detectors for HEP. The dark noise levels of these quantum non-demolition photon detectors can be orders of magnitude below those of detectors based on even quantum-limited linear amplifiers. An application is already envisioned in which qubit-based photon detectors may enable the next generation of dark matter axion experiments that would search for higher-mass axions. Moreover, the several-gigahertz operating frequency of superconducting qubit devices coincides with the frequencies of superconducting radio frequency (SRF) cavities used in HEP. Thus, the large investment by HEP in SRF cavity materials processing and fabrication technologies can potentially be applied to improve the performance of qubit devices by orders of magnitude, possibly providing significant improvements in quantum computing.
Fermilab has engaged local experimental quantum computing and superconducting materials experts and is constructing two milli-Kelvin test stands to enable this technology transfer. One test stand will incorporate a high-field magnet to explore the axion detector option, and the other will be dedicated to studies of superconducting materials at ultralow temperatures where thermal noise can be exponentially suppressed. Both efforts have received LDRD seed funding and will proceed over the next 2-3 years.
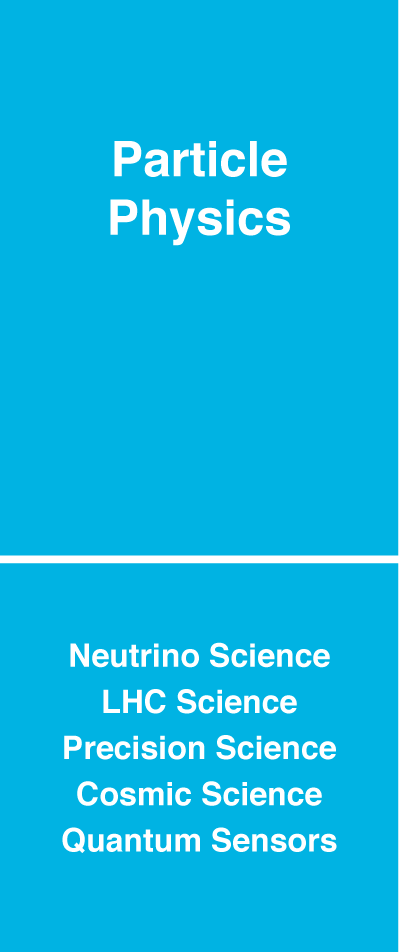